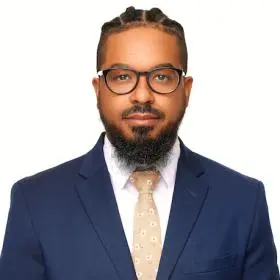
Fill This Form To Receive Instant Help
Words: 3540
Published: May 30, 2024
Thermodynamic processes that occur spontaneously are all irreversible; that is, they proceed naturally in one direction but never reverse. A rolling wheel across a rough road converts mechanical energy into heat due to friction. The former is irreversible, just as it is impossible that a wheel at rest would spontaneously start moving and getting colder as it moves instead.
In this paper, the second law will be introduced by considering several thermodynamic devices: (1) heat engines, which are partly successful in converting heat into mechanical work, and (2) refrigerators and heat pumps, which are partly successful in transferring heat from cooler to hotter regions.
Heat Engines
The essence of our technological society is the ability to utilize energy resources other than muscle power. These energy resources come in many forms (e.g. solar, geothermal, wind, and hydroelectric). But even though we have a number of them available in the environment, most of the energy used for machinery comes from burning fossil fuels. This process yields heat, which then can be directly used for heating buildings in frigid climate, for cooking and pasteurization, and chemical processing. But to operate motors and machines, we need to transform heat into mechanical energy.
Any device that converts heat partly into mechanical energy or work is called a heat engine. They absorb heat from a source at a relatively high temperature, i.e. a hot reservoir (like combustion of fuel), perform mechanical work, and discard some heat at a lower temperature (Young & Freedman, 2019). In correspondence to the first law of thermodynamics, the initial and final internal energies of this system are equal when carried through a cyclic process, as in
Thus, we can say that net heat flowing into the engine in a cyclic process is equal to the net work done by the engine (Brown et al., 2017).
We can illustrate how energy is transformed in a heat engine using the energy-flow diagram (Fig. 1). The engine itself is represented by the circle. The amount of heat QH supplied to the engine by the hot reservoir is directly proportional to the width of the incoming “pipeline” at the top of the diagram. The width of the outgoing pipeline at the bottom is proportional to the magnitude |QC| of the heat discarded in the exhaust. The branch arrow to the right represents the portion of the heat supplied that the engine converts to mechanical work, W.
When an engine repeats the same cycle over and over, QH and QC represent the quantities of heat absorbed and rejected by the engine during one cycle; QH is positive, and QC is negative. The net heat Q absorbed per cycle is
= + =||−||
The useful output of the engine is the net work W done by the working substance. From the first law,
= = + =||−||
Ideally, we would like to convert all the heat QH into work; in that case we would haven QH = W and QC = 0. Experience shows that this is impossible; there is always some heat wasted, and QC is never zero. We define the thermal efficiency of an engine, denoted by e as the quotient
The thermal efficiency e represents the fraction of QH that is converted to work. To put it another way, e is what you get divided by what you pay for. This is always less than unity, an all-too-familiar experience! In terms of the flow diagram of Fig. 1, the most efficient engine is one for which the branch pipeline representing the work output is as wide as possible and the exhaust pipeline representing the heat thrown away is as narrow as possible.
When we substitute the two expressions for W given by Eq. 1.2 into Eq. 1.3, we get the following equivalent expressions for e:
Refrigerator and Heat Pump
We can understand the mechanism of a refrigerator as opposed to a heat engine. As explained in the first part, a heat engine takes heat from a hot reservoir and gives it off to a colder place. A refrigerator operates in reverse, i.e. it takes heat from a cold place (inside of the refrigerator) and gives off that heat into a warmer place, often the surrounding air in the room where the refrigerator is located. In addition, while a heat engine has a net output of mechanical work, the refrigerator requires a net input of mechanical work (Poredoš, 2021).
Fig 2.1 shows an energy-flow diagram for a refrigerator. From the first law of thermodynamics for a cyclic process,
+−=0 −=− or because both QH and W are negative,
||= +| |
It only shows that the heat |QH| given off from the working substance and given to the hot reservoir is always greater than the heat QC taken from the cold reservoir.
From an economic point of view, the most efficient refrigeration cycle is one that takes off the greatest amount of heat |QC| from inside the refrigerator for the least use of mechanical work, |W|. The relevant ratio is |QC|/|W|, called the coefficient of performance, K, which implies that the larger this ratio is, the better the refrigerator.
A variation on this is the heat pump, which functions like a refrigerator, but turned inside out. A heat pump is used to heat buildings by cooling the air outside. The evaporator coil is placed outside, as it takes heat from cold air, while the condenser coils are inside, which gives off heat to the warmer air. In this design, the heat |QH| taken inside a building can be considerably greater than the work |W| needed to get it there.
Conclusion
In the bottom line, it is impossible to create a heat engine that completely converts heat to work, i.e. 100% thermal efficiency. It only corresponds to the second law of thermodynamics which states that it is impossible for any system to undergo a process in which it absorbs heat from a reservoir at a single temperature and converts the heat completely into mechanical work, with the system ending in the same state in which it began. Heat flows spontaneously from hotter to colder objects, never the reverse. A refrigerator does take heat from a colder to a hotter object, but its operation requires an input of mechanical energy or work. We can deduce that it is impossible for any process to have as its sole result the transfer of heat from a cooler to a hotter object.
References
Brown, T. L., LeMay, Jr., H. E., Bursten, B. E., Murphy, C. J., Woodward, P. M. (2017, January 1). Chemistry: The Central Science (14th ed.). Pearson.
Ozerov, R. P., & Vorobyev, A. A. (2007). 3 - Molecular Physics. Physics for Chemists. (), 169–250. https://doi.org/10.1016/B978-044452830-8/50005-2
Poredoš, A. (2021, April 25). Thermodynamics of Heat Pump and Refrigeration Cycles. Entropy, 23(5), 524. https://doi.org/10.3390/e23050524
Young, H. D., & Freedman, R. A. (2019). University Physics with Modern Physics (15th ed.). Pearson.
Keep in mind: This sample was shared by another student.